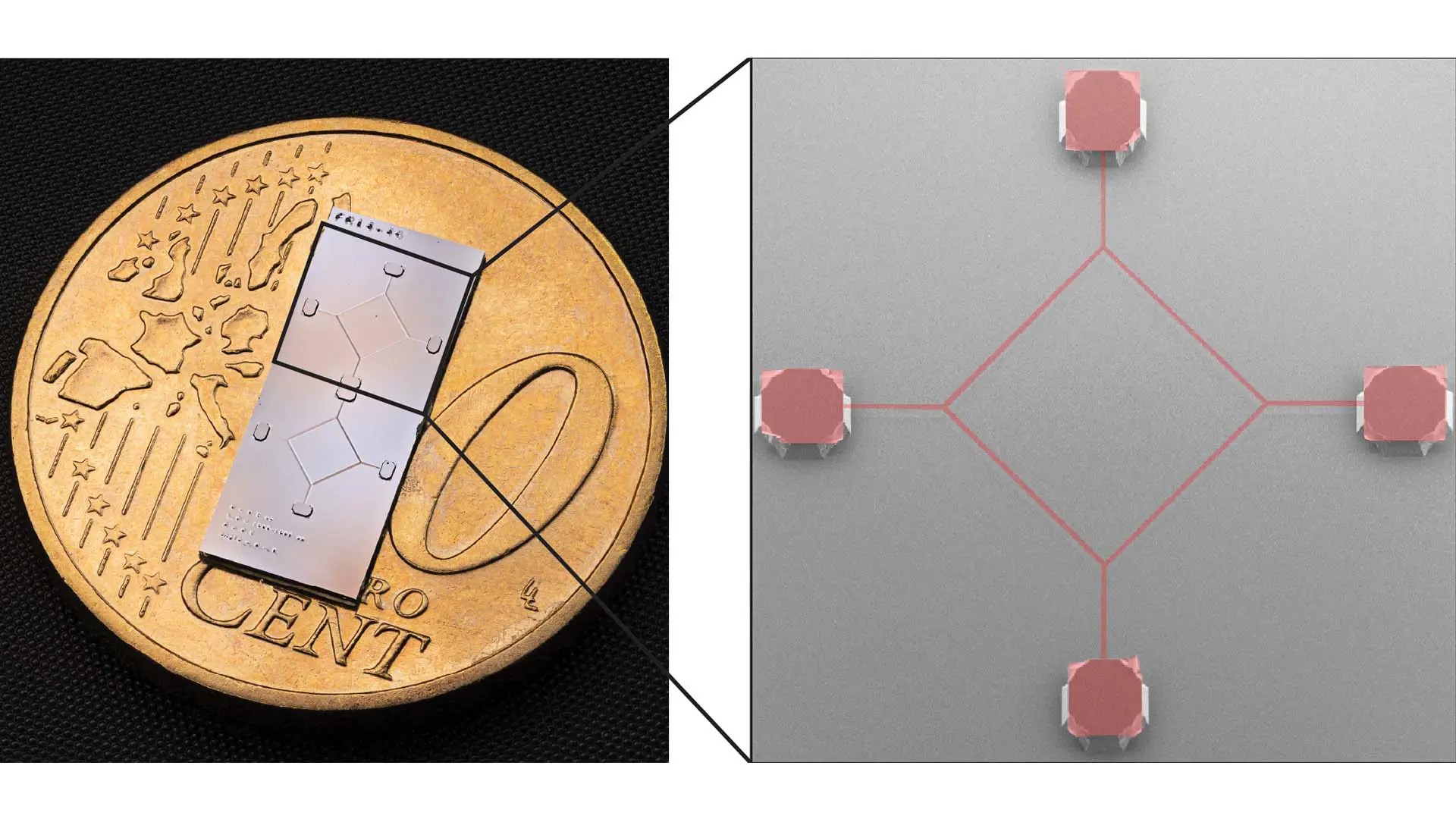
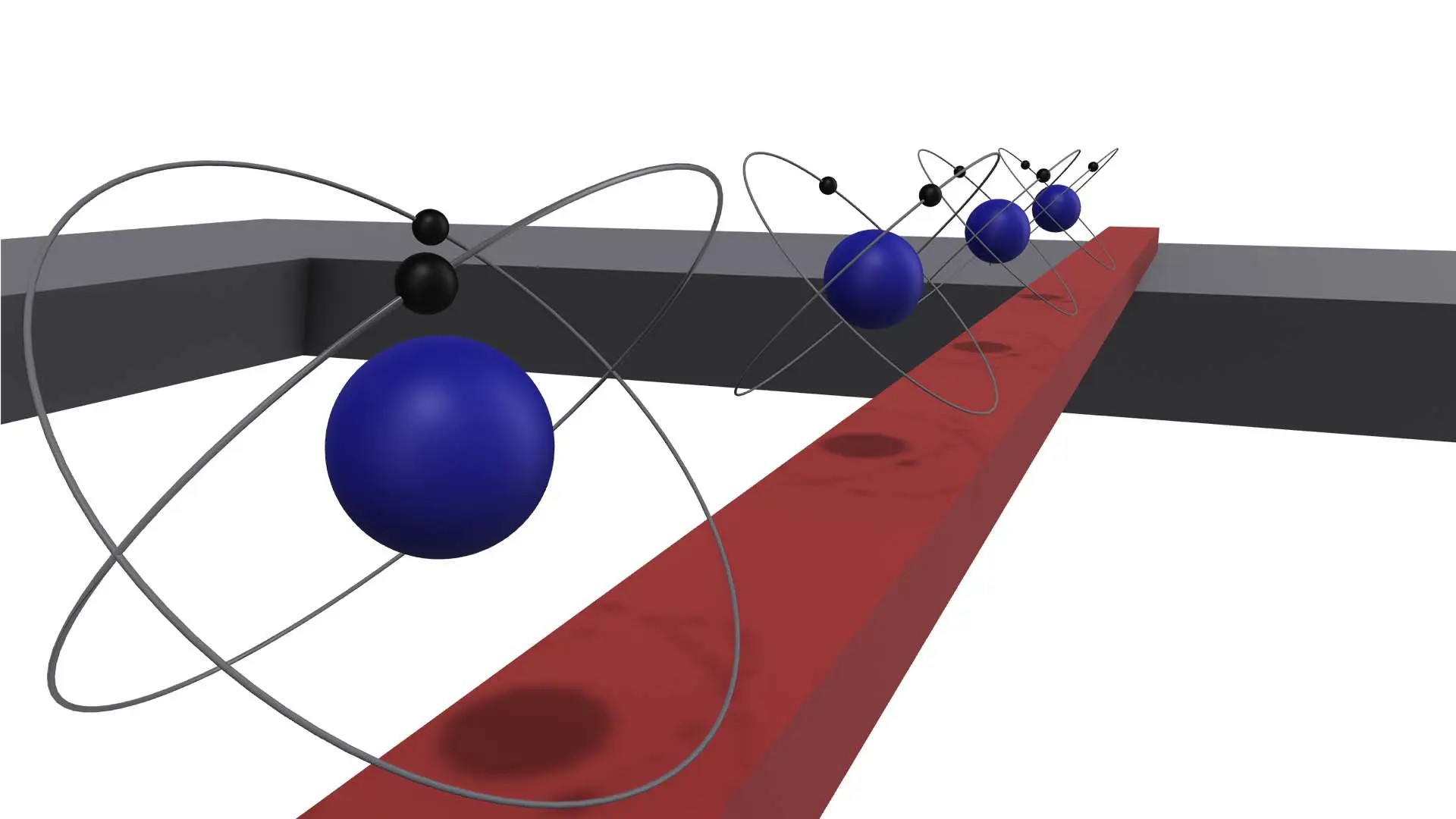
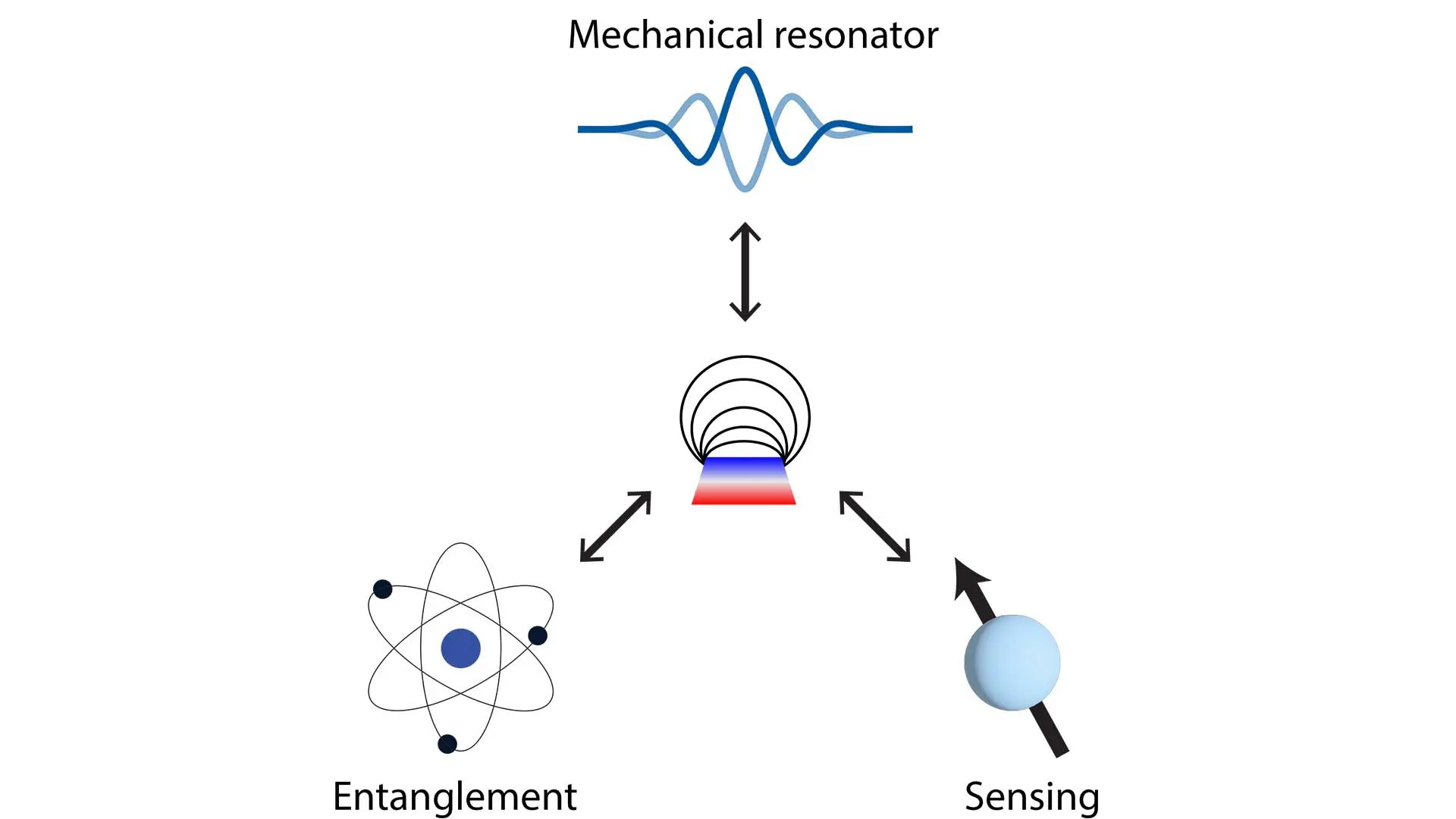
The Hybrid Quantum Systems Laboratory (HQL) makes interfaces between cold atoms, photonic integrated circuits and ultrahigh-Q mechanical resonators. The main goals of the research group is to generate long-lived quantum states of motion in macroscopic mechanical resonators, create quantum sensors with improved precision compared to the state of the art and study light-matter interactions in new paradigms using nanophotonics.
Functionalization of mechanical resonators to create quantum states of motion and spin sensors
Suspended mechanical resonators of silicon nitride are excellent sensors for small forces and exhibit the highest mechanical quality factors of any mechanical resonator at room temperature. Functionalization with nanomagnets on these mechanical resonators makes them sensitive to small magnetic forces, which would allow direct magnetic coupling to trapped cold atoms. In this scenario, you can use the internal states of a single atom trapped in an optical tweezer to create nonclassical states of motion. The main aim of this direction is to realize an optomechanical system that can be used to control and read out the motion of a mechanical resonator with an implanted nanomagnet. This system will then be used both for spin sensing and quantum engineering.
Cold atoms coupled to photonic integrated circuits
Computers use different components to process, store and transmit information. Similarly, hybrid quantum systems exploit the strengths of different quantum systems in an integrated whole. For example, light can transmit quantum information over long distances with low loss while neutral atoms and solid state spins are excellent quantum memories. Photonic integrated circuits, where light is routed and manipulated on photonic chips, are a particularly promising platform for creating hybrid quantum systems by coupling multiple systems to light. Strontium atoms are used in the best atomic clocks and show promise for quantum computing, but have yet to be coupled to photonic integrated circuits.
We will use the flexibility of silicon nitride photonics to realize strong and novel interactions between atoms and light by fabricating tailored photonic structures. As an example, while atoms usually emit light in random directions, so-called chiral waveguides can enforce directional atomic emission—a key capability for future quantum networks. These photonic structures will also support superradiant lasing, which will be used in the next generation of ultrastable lasers. Light-mediated atom-atom interactions can be used to study quantum many-body physics and to generate entangled quantum states that improve the sensitivity of atomic sensors.
More about The Hybrid Quantum Systems Laboratory (HQL)
The HQL is a new laboratory started by Nils Johan Engelsen who previously developed ultrahigh-Q mechanical resonators and did quantum optomechanics in Tobias Kippenberg’s group at EPFL. He got his PhD at Stanford University with Prof. Mark Kasevich, where he generated entangled states of Rubidium atoms using optical cavities. You can find a full publication list on Nils Johan Engelsen's Google Scholar and below are a selection of his publications:
1. M. J. Bereyhi, A. Beccari, R. Groth, S. A. Fedorov, A. Arabmoheghi, T. J. Kippenberg, N. J. Engelsen, Hierarchical tensile structures with ultralow mechanical dissipation, Nature Communications 13, 3097 (2022)
2. M. J. Bereyhi, A. Arabmoheghi, A. Beccari, S. A. Fedorov, G. Huang, T. J. Kippenberg, N. J. Engelsen, Perimeter modes of nanomechanical resonators exhibit quality factors exceeding 10¬¬9 at room temperature, Physical Review X 12, 021036 (2022)
3. A. H. Ghadimi*, S. A. Fedorov*, N. J. Engelsen*, M. J. Bereyhi, R. Schilling, D. J. Wilson, T. J. Kippenberg, Elastic strain engineering for ultralow mechanical dissipation, Science 360, 764–768 (2018) *Indicates equally contributing author
4. N. J. Engelsen, R. Krishnakumar, O. Hosten, M. A. Kasevich, Bell correlations in spin-squeezed states of 500 000 atoms, Physical Review Letters 118, 140401 (2017)
5. O. Hosten, R. Krishnakumar, N. J. Engelsen, M. A. Kasevich, Quantum phase magnification, Science 362, 6293, 1552-1555 (2016)
6. O. Hosten, N. J. Engelsen, R. Krishnakumar, M. A. Kasevich, Measurement noise 100 times lower than the quantum-projection limit using entangled atoms, Nature 529, 505–508 (2016)