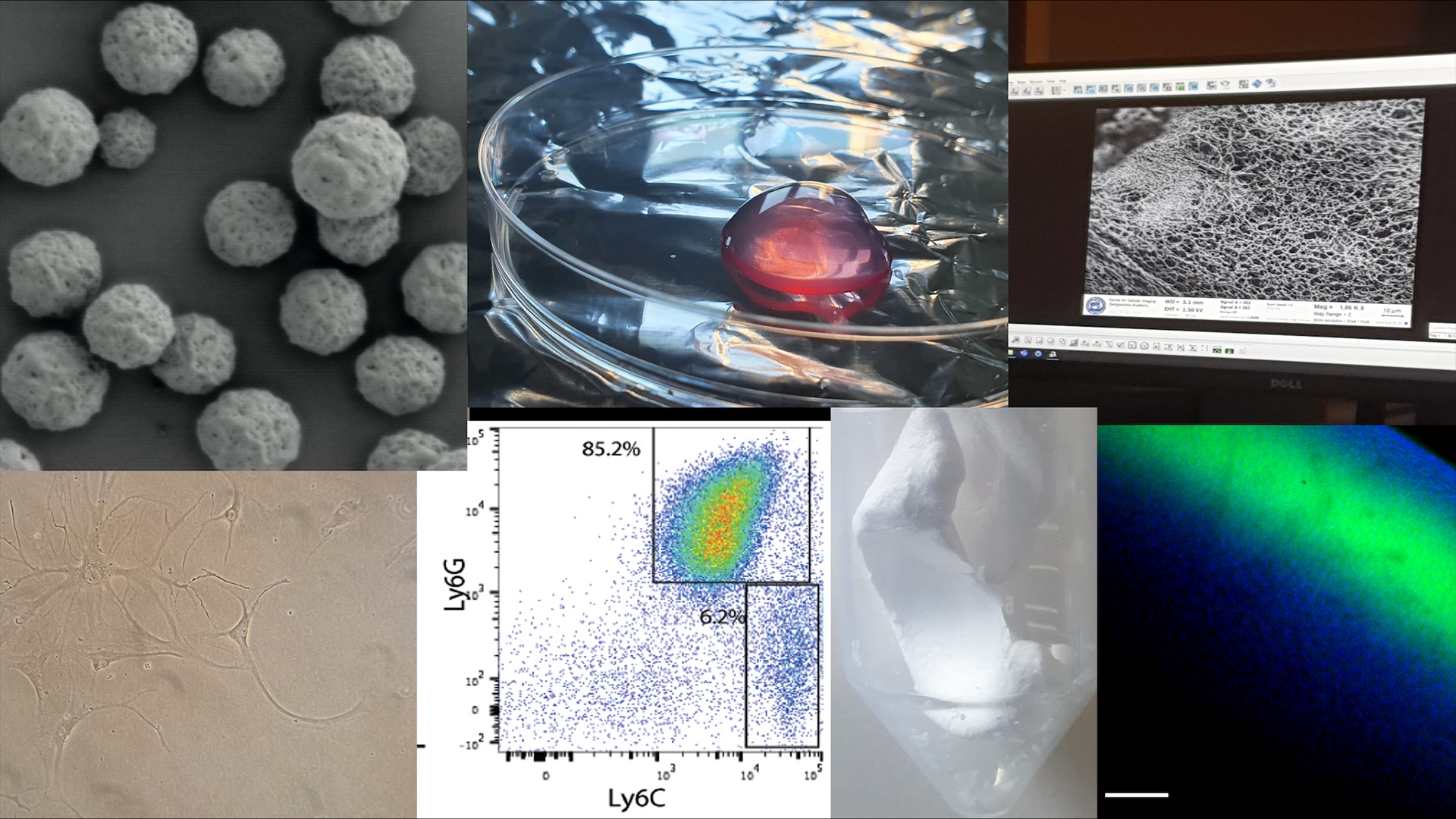
The goal of any successful therapy should be to improve clinical outcomes and ultimately restore normal tissue function. In Alexandra Stubelius’ research team, we study disease mechanisms and develop nanosystems and materials that take advantage of immune functions to target drug delivery and reshape cell signaling. As the immune system and inflammation contribute to a wide variety of disorders, including cancer and autoimmune diseases, these technologies can contribute and improve the health and quality for a broad range of patients.
Focus Area Overview
Our research focuses on the interactions between polymeric nanoparticles and biomaterials with the disease microenvironment, where we are particularly interested in interactions with the immune system. We mainly base our materials on the many functions of saccharides and modified polysaccharides- either as material base or as our targets. To design these materials, we combine the knowledge of pharmaceutical scientists, immunologists, bio-, chemical-, nano-, and material engineers, and clinicians.
By delicately fine-tuning materials’ properties towards their intended purposes, tissue environments, and physical properties, we regulate immune responses to achieve improved health outcomes.
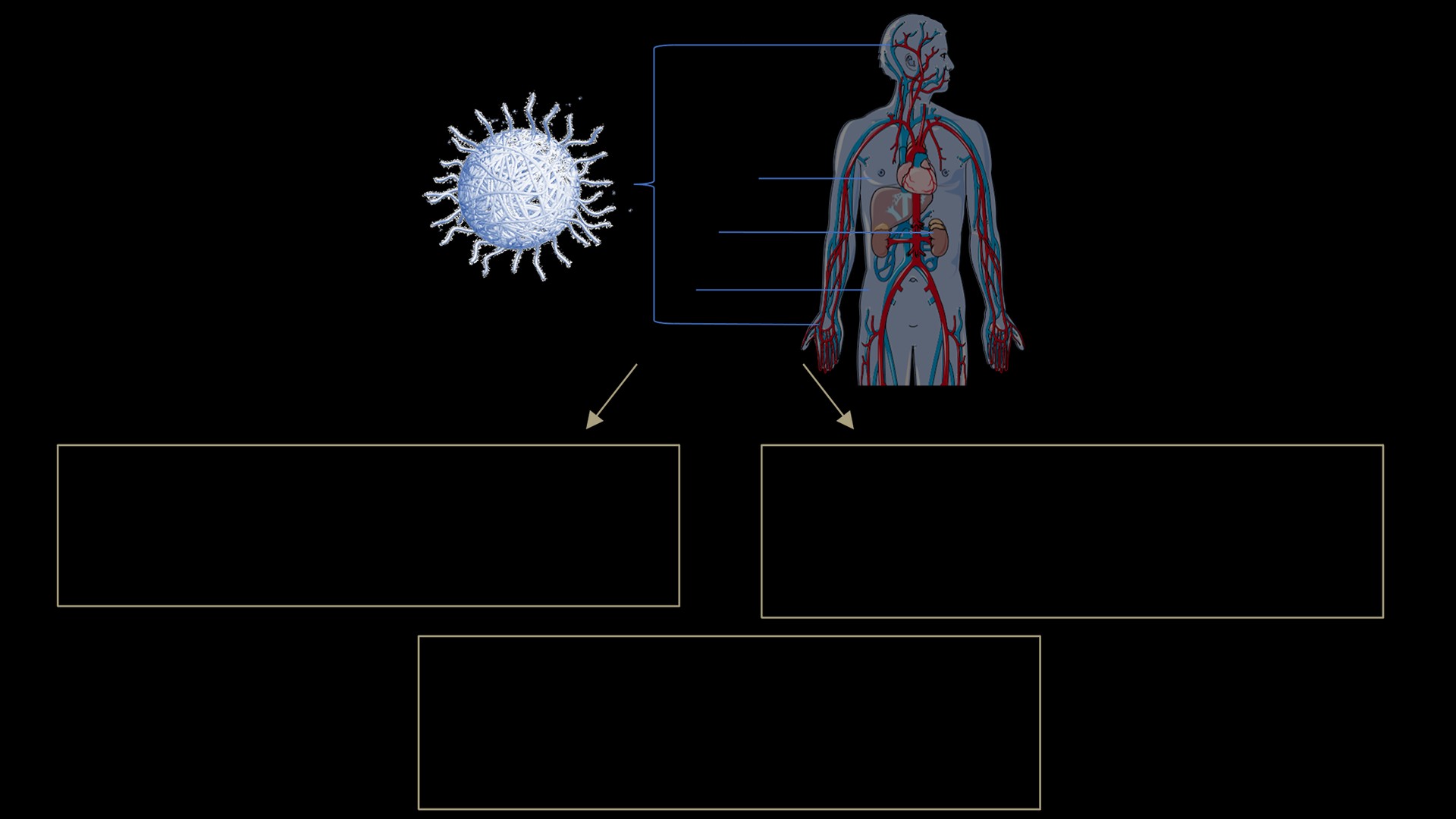
Increase Drug Delivery Specificity to Reduce Side Effects
Design Inflammation-Specific Nanocarriers controlled by the microenvironment
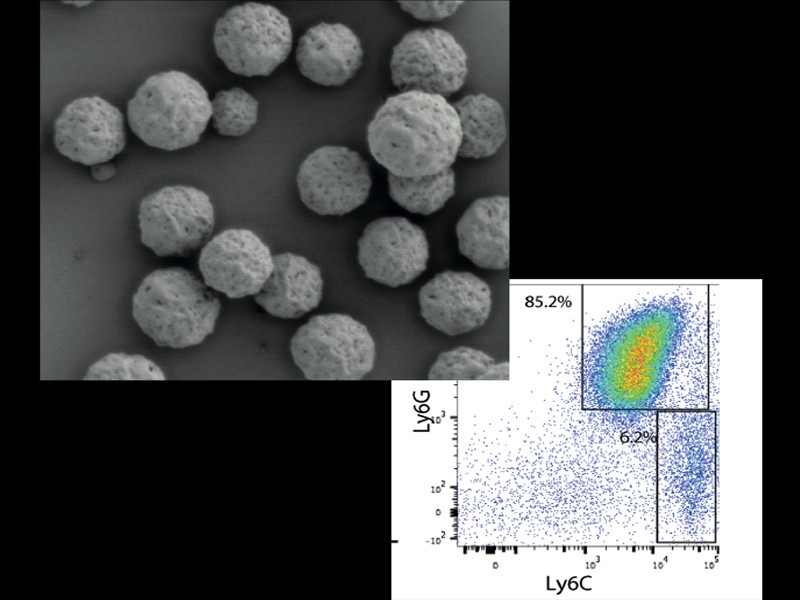
One of the most pressing challenges in modern medicine is the precise delivery of treatments and drugs to their intended targets within the body. This is crucial because many drugs, even those with promising therapeutic potential, can suffer from drawbacks such as rapid elimination from the bloodstream, toxicity when reaching unintended locations, or difficulty in dissolving and penetrating tissues. A key approach involves employing smart materials that can selectively deliver drugs to specific disease sites, minimizing systemic exposure and maximizing therapeutic impact.
To achieve effective inflammation reduction with minimal drug dosage, we use both conventional anti-inflammatory drugs or repurpose drugs in combination with novel smart materials. This approach holds promise for delivering targeted, personalized, and more efficient therapeutic interventions for a range of inflammatory conditions.
Design Materials to Modulate Key Immunecell Checkpoints
Targeting Glycans As Novel Mechanisms
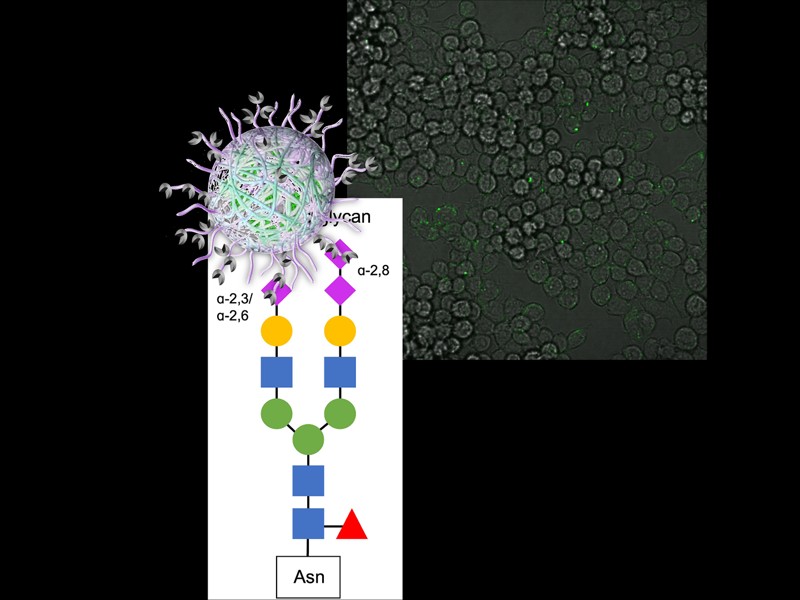
A fundamental aspect of immune cell communication lies in the intricate network of sugar molecules, known as the glycans, that adorn proteins, lipids, and cells. These sugar structures play a crucial role in orchestrating immune responses, facilitating interactions between various immune cells and guiding their functions.
By investigating and harnessing the signaling capabilities of glycans, we seek to develop novel therapeutic and detection approaches that can more precisely regulate immune cell activity. Unlike conventional medications that often involve broad inhibition of immune responses, our approach aims to target specific sugar components, enabling a more nuanced modulation of immune functions.
Our approach involves a synergistic combination of nanotherapeutics and extracellular matrix (ECM)-based strategies to enhance the development of biomaterials for medical applications.
Define How Immune-cells, Nanoparticles and Tissues Interact
Develop Increasingly Specific Models and Immunomodulatory Materials
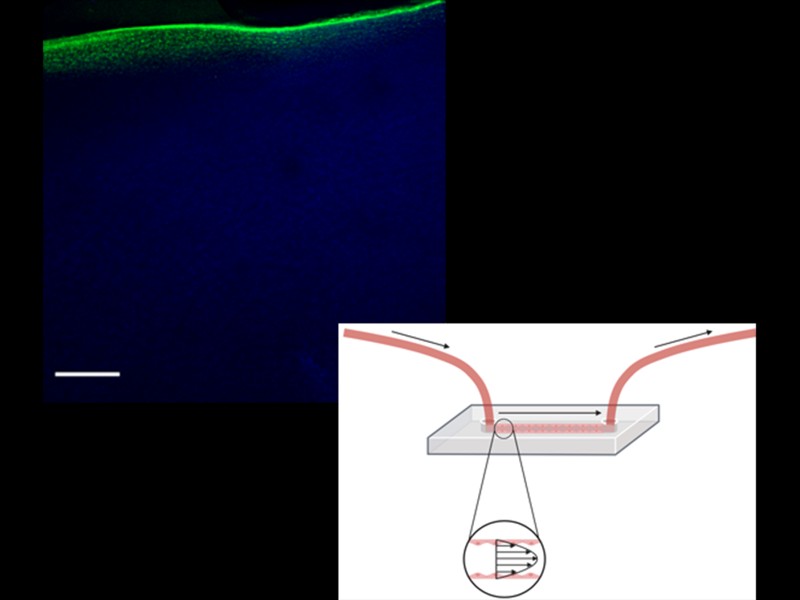
To ensure that materials and biological systems interact effectively and elicit the desired therapeutic effects, we develop enhanced model systems and extensively evaluate material-biology interactions. When materials encounter the body's intricate biological milieu, they undergo a transformative process, shedding their "synthetic identity" and adopting a "biological identity." This dynamic adaptation significantly influences the subsequent physiological response.
By meticulously modeling and analyzing these material-biology interfaces, we can gain valuable insights into how materials behave within the body. This knowledge empowers us to predict with greater accuracy whether a developed material will fulfill its intended purpose, potentially be prematurely eliminated, or inadvertently trigger adverse effects.
Our research focuses on two key areas of application: blood vessel mimetics and joint drug delivery. We strive to develop novel vessel-on-a-chip models that can mimic the intricate structure and function of natural blood vessels, enabling enhanced modeling of nanoparticle delivery. In the domain of joint drug delivery, we aim to understand how to better design delivery vehicles that selectively deliver therapeutic agents to the affected joint spaces, minimizing systemic exposure and maximizing local efficacy.
Equipment and Instruments
Student Projects and Vacancies
- Potential Amanuensis students, Master thesis students and Summer interns that are interested in doing research in the lab please contact Alexandra Stubelius.
- Are you interested in joining the group as a PhD student? One way is to apply for the annual Excellence PhD student positions within AoA Nano at Chalmers (or check Chalmers’ Vacancies).
- If you are interested in jointly applying for a fellowship to support your stay in the group please contact Alexandra Stubelius.