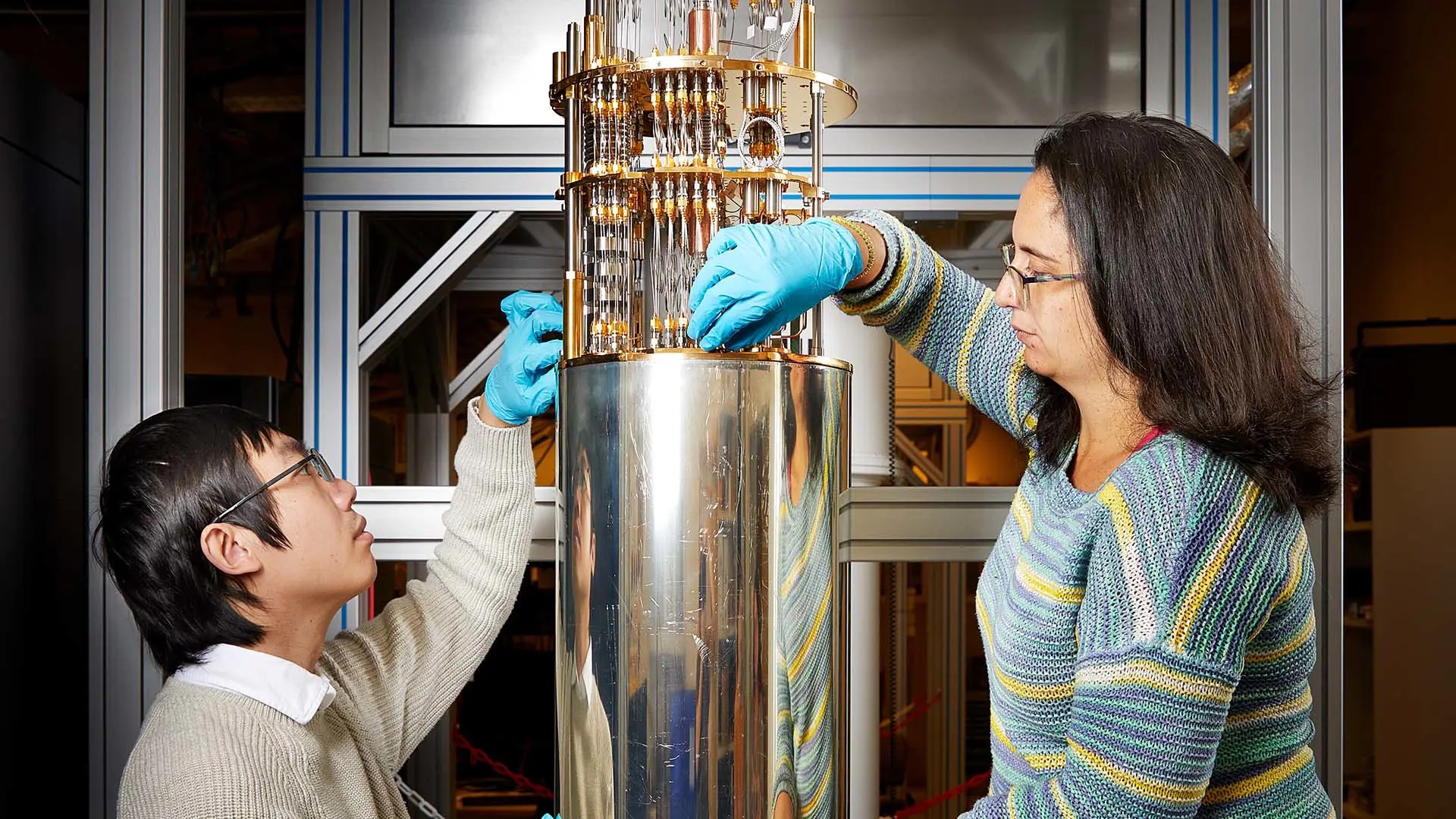
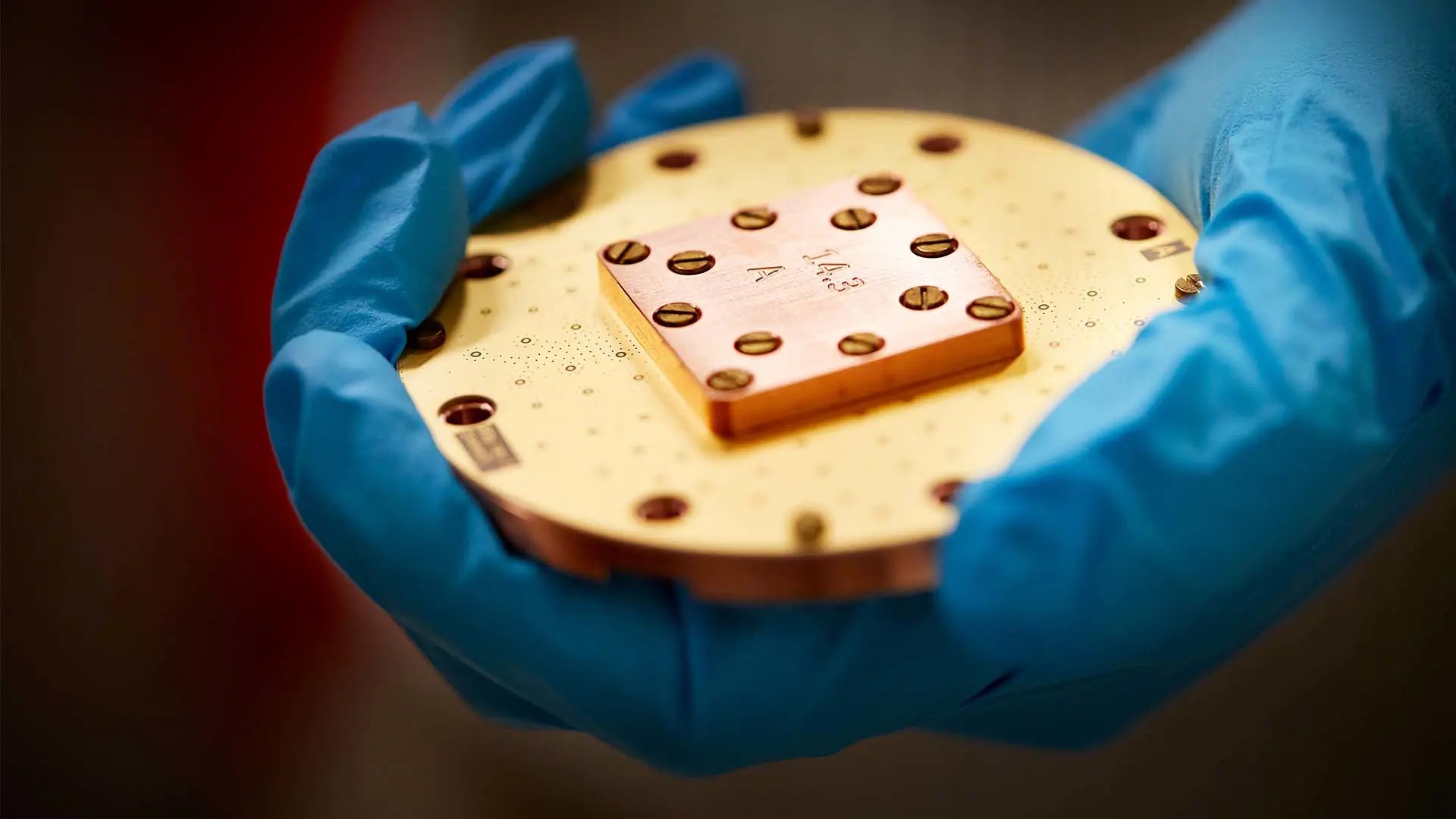
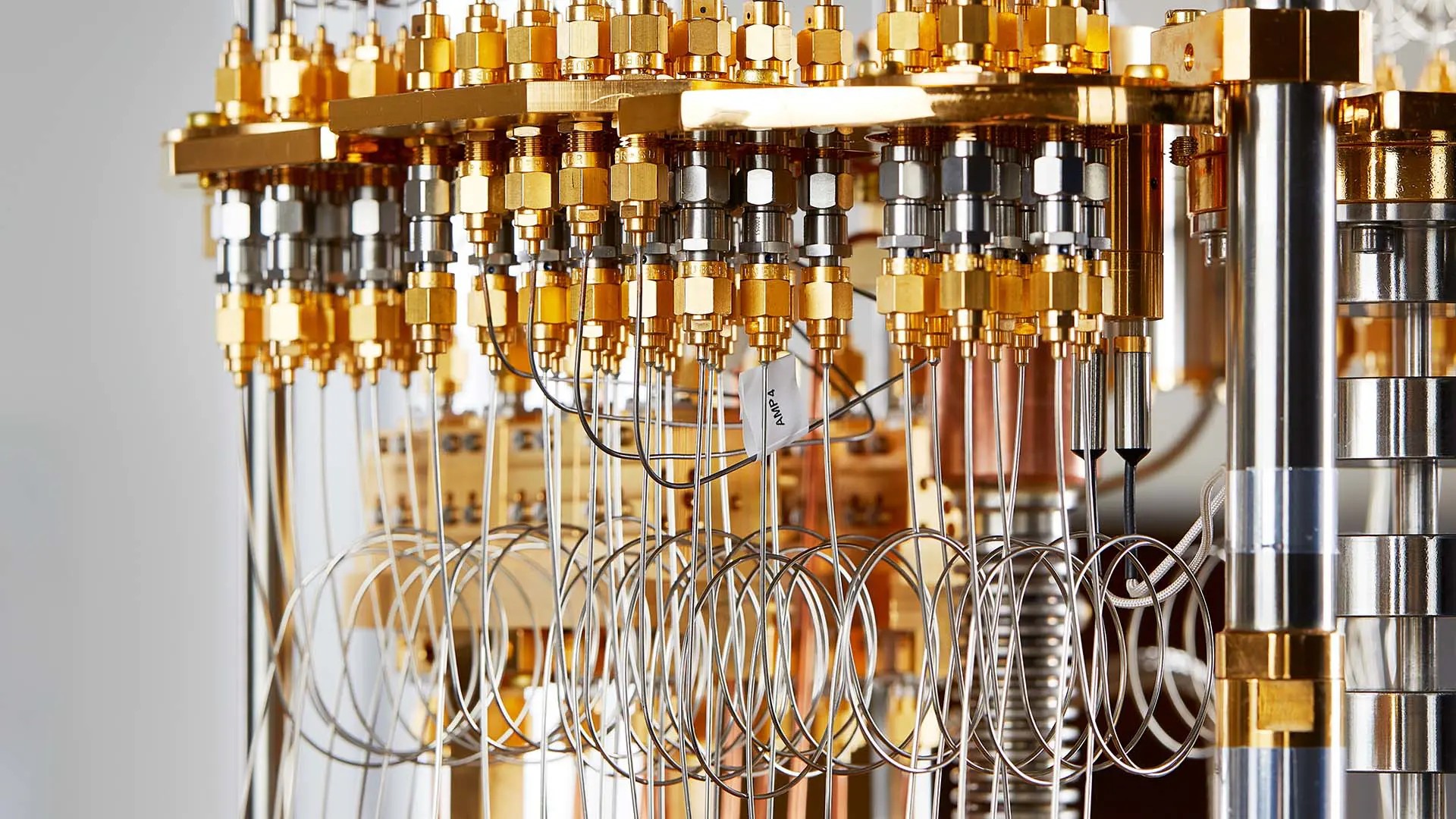
The core project within the Wallenberg Centre for Quantum Technology is to build a superconducting quantum computer with one hundred well-functioning qubits and to run at least one useful algorithm.
This page describes quantum computing research performed within WACQT. For a general introduction to quantum computing, please go to the page Quantum computing.
Quantum computing with superconducting circuits
WACQT’s core project quantum computer is being developed in a targeted project at Chalmers. It is based on superconducting circuits – a technology with which Chalmers’ team has extensive experience and which also is one of the main platforms for quantum computing pursued around the world. Some advantages of this technology are:
- The superconducting circuits that constitute the qubits can be designed and manufactured on a chip, similar to an ordinary microchip. The manufacturing method allows for scaling up to a large number of qubits and provides flexibility in how to inter-connect them.
- Superconducting qubits are controlled and manipulated using microwaves – a frequency band in which a lot of good equipment is already available.
- Quantum-logical operations can be performed very quickly.
We are pursuing two paths in parallel: one more mainstream multi-qubit processor approach and one newer, more unique continuous-variable approach. They use similar technology, hardware, and control system and therefore benefit from each other.
Multi-qubit quantum processor
In our most developed approach, we are working on a multi-qubit quantum processor. In this approach, information is encoded in qubits which have two quantum states representing zero and one. Each qubit is constituted by a lossless, resonant circuit which includes a so-called Josephson junction. Such a circuit – though large enough to be visible to the naked eye – behaves as a single atom and exhibits all quantum properties such as superposition, entanglement etc.
Our strategy has been to first get our qubits and quantum-logic gates to work really well on a small scale, before scaling up. Therefore, we spent the first years of WACQT working with a quantum processor chip with only two qubits. In 2019, we achieved the desired performance, and also demonstrated that we can map and run a useful algorithm on the processor, as described in Small-scale demonstration of useful quantum algorithm.
Now we have begun to scale up in fairly large steps. We have developed a 3D chip design, that forms the foundation for our new 25-qubit chip and which is scalable towards 50–100 qubits. Learn more about the 3D architecture in this short film by Hang-Xi Li.We have also developed a full software stack, which gives our theorists online access to one of our quantum processors to test run quantum algorithms.
Continuous-variable quantum computing
In the second of our two parallel paths, we explore using superconducting cavities containing microwave fields for continuous-variable quantum computing. The key difference to the multi-qubit approach is that information is not stored in superconducting qubits, but instead in non-classical oscillation states in the cavities. Hear Mikael Kervinen explain the difference in this short film. However, superconducting qubits are still used to access those states, and to manipulate and read out the information.
This is a newer approach which promises longer lifetimes and easier correction of errors compared to the multi-qubit approach. However, the scaling-up is not really solved yet.
Making the machines useful
An important task for our theory team is to explore what a relatively small quantum computer could do and try to find a "killer application" – that is to find the first useful problems, that are beyond the reach of regular computers, that a relatively small quantum computer can solve. We work on this in collaboration with our industrial partners in fields like drug development, logistic optimization and in the near future also genomics. Building on a very close interaction between hardware and software development teams in a so-called co-design loop, we will squeeze the most useful quantum power possible out of the growing quantum computer.
Rydberg ion quantum computing
WACQT also includes a quantum computer project based on Rydberg ions at Stockholm University. In this approach, we are working on a quantum processor based on ions that are trapped – that is, held in place – by electric fields. The qubits are encoded in the ions’ electronic state and qubit operations are performed by laser pulses. For two-qubit operations the electrons of the ions are laser-excited to high orbitals, so-called Rydberg states, which allows for much faster gates than previously possible in ion-trap systems. This Rydberg state approach was pioneered by the Stockholm group. Read more in Speeding-up quantum computing using giant atomic ions.
European collaboration
October 2018 to March 2022, WACQT’s quantum computing team was part of OpenSuperQ – a large European effort to build a superconducting quantum computer and make it available in the cloud (read more in Joining forces for a European quantum computer).
A continuation was proposed and received funding. In 2023, project the project was expanded from 10 to 30 partners and renamed OpenSuperQPlus100 as it targets functional 100-qubit quantum computers. Compared to the previous phase, it has now stronger focus on engineering.
“The project is entirely aligned with the quantum hardware and software goals of WACQT,” says Jonas Bylander, one of the principal investigators for Chalmers.
WACQT research leaders in quantum computing
Leaders of theoretical efforts in quantum computing:
Göran Johansson, goran.l.johansson@chalmers.se, +46 31 772 3237
Guilia Ferrini, ferrini@chalmers.se, +46 31 772 6417 (focus on continuous-variable)
Leaders of experimental efforts in superconducting quantum computing:
Per Delsing, per.delsing@chalmers.se, +46 31 772 3317
Jonas Bylander, jonas.bylander@chalmers.se, +46 31 772 5132 (focus on multi-qubit)
Simone Gasparinetti, simoneg@chalmers.se, +46 31 772 65 73 (focus on continuous-variable)
Giovanna Tancredi, tancredi@chalmers.se, +46 31 772 1394 (focus on multi-qubit)
Leader of efforts in Rydberg ion quantum computing:
Markus Hennrich, markus.hennrich@fysik.su.se , +46 8 553 786 14