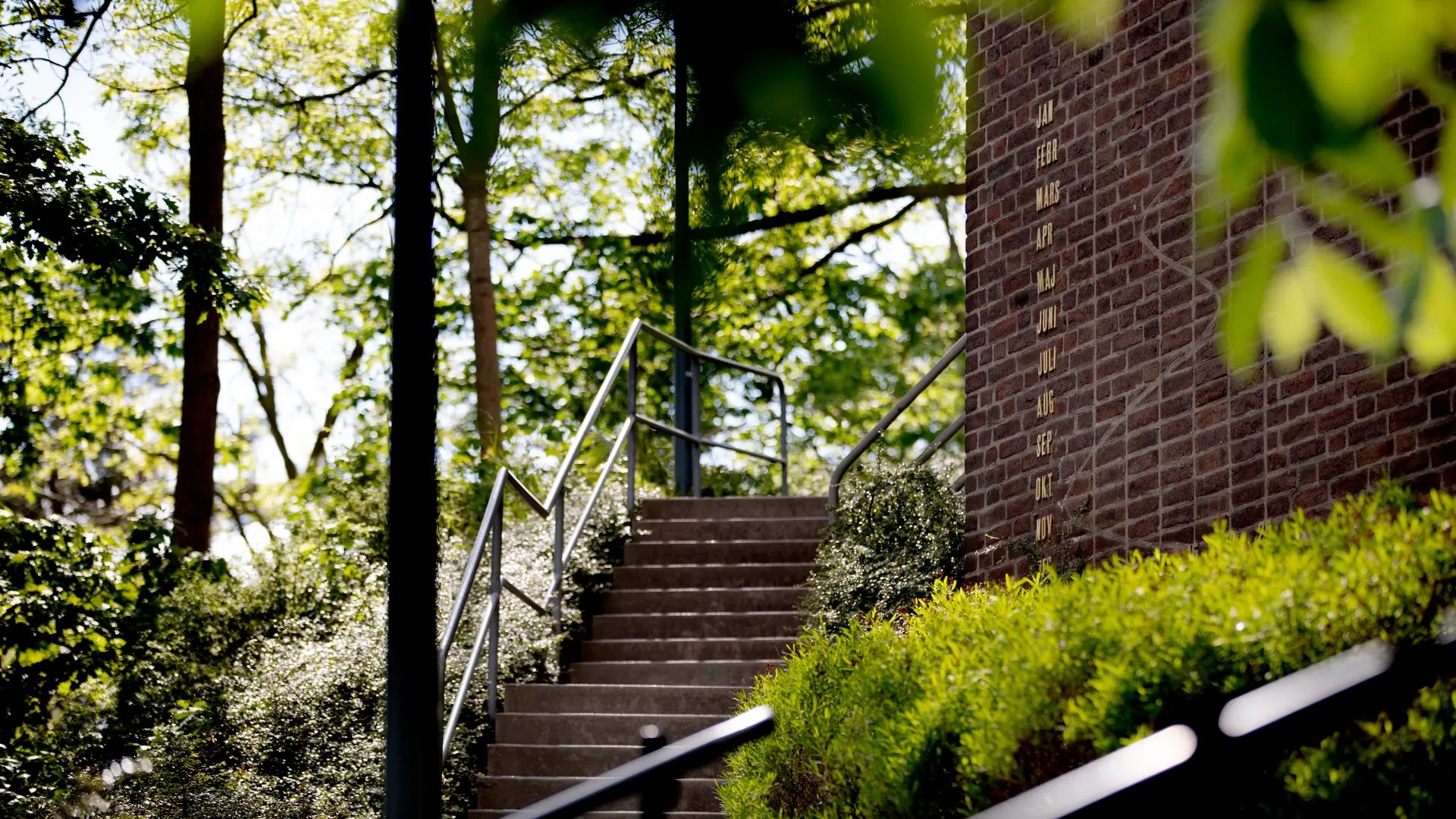
The Gothenburg Physics Centre (GPC) coordinates cooperation between four departments: Physics, Space, Earth and Environment, Microtechnology and Nanoscience at Chalmers University of Technology, and Physics at the University of Gothenburg. Our overreaching aim is to promote the subject of physics in Gothenburg by a wide variety of initiatives.

NORNDiP 2025
Welcome to the conference Nordic Network for Diversity in Physics that will take place at Chalmers, November 18-19 2025.
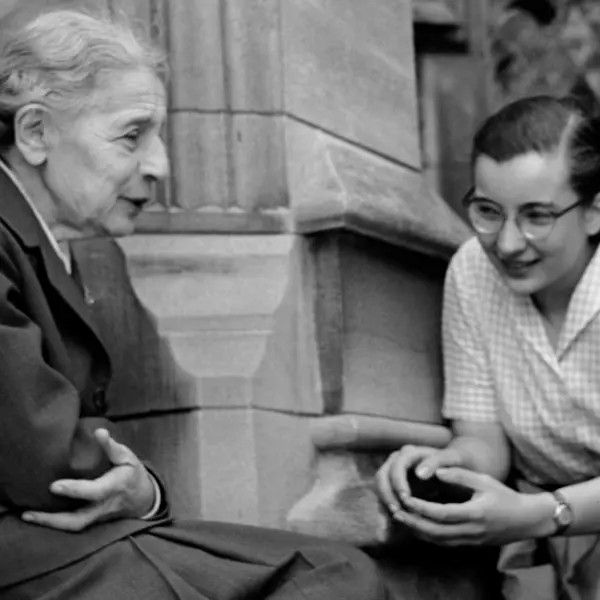
Gothenburg Lise Meitner Award
The Gothenburg Lise Meitner Award is awarded by the Gothenburg Physics Centre to a scientist who has made a breakthrough discovery in physics.

European Physics Olympiad 2026
In June 2026, the 10th European Physics Olympiad, EuPhO, will be held in Gothenburg, with Chalmers University of Technology and the University of Gothenburg as hosts.
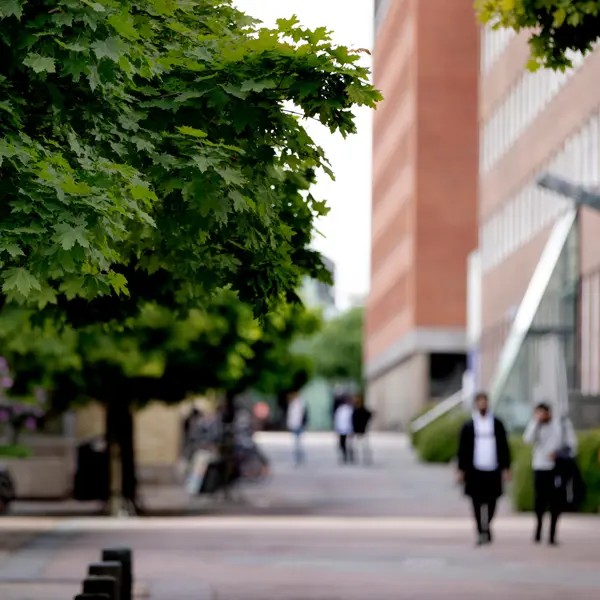
Meet some of our researchers
Meet some of the prominent researchers who are part of Gothenburg's physics community.