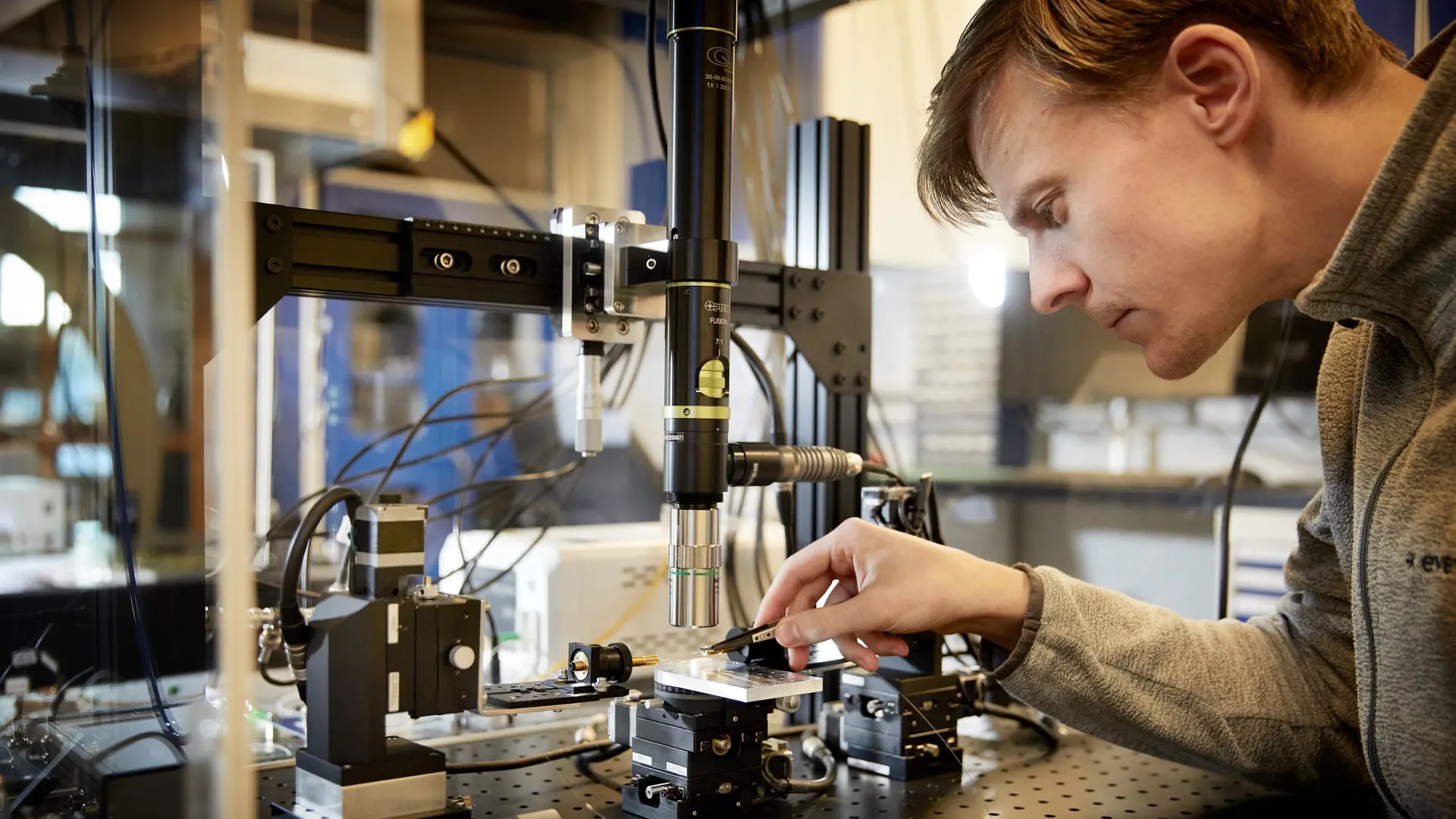
Interconnecting remote quantum computers may accelerate the development of quantum technologies. But the road there is paved with several challenges. Now a research team at Chalmers University of Technology has made progress towards solving a central problem in the area - and thus taken a step closer to the possibility of interconnecting quantum computers using light.
Quantum computers around the world are becoming increasingly powerful – one qubit at the time. The WACQT quantum computer at Chalmers University of Technology has currently reached 25 qubits and is aiming for 100 superconducting qubits. Developing quantum computers takes time and requires considerable resources, which calls for alternative approaches. At the Division of Quantum Technology at Chalmers University of Technology, researchers are hence exploring the possibility of connecting remote quantum computers with each other to make better use of the total computing power.
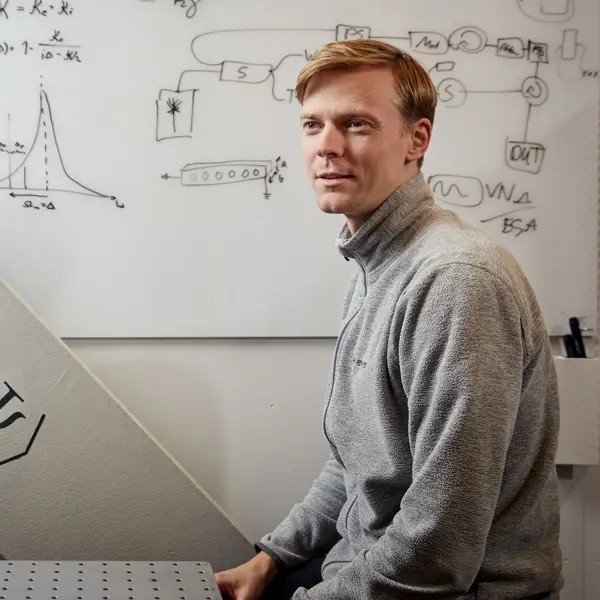
“It’s not sustainable in the long run to continue scaling up quantum computers within one cryostat. By instead interconnecting quantum computers via light, we may achieve an increased processing power using several smaller units that are already available,” says Johan Kolvik, doctoral student at the Division of Quantum Technology at the Department of Microtechnology and Nanoscience.
Using light to avoid noise
However, using microwaves - which is the native language of superconducting qubits - to transmit signals between quantum computers comes with a well-known problem. The sensitive quantum signals are drowned and lost in the thermal noise associated with microwave transmission at room temperature. In recent years, researchers have therefore started to explore the possibility of using light as a low-noise and high-bandwidth information carrier – much like in classical datacenters.
To optically connect quantum computers, we need devices to transmit the quantum computer’s microwave signals into the optical network. Using acoustics as an intermediary promises to be a solution with sufficiently high bandwidth and especially low dissipated energy. The possibility to optically read out a qubit has been around since recently, however, the links used today are not yet able to effectively and bidirectionally connect remote quantum computers with each other. A central challenge for this type of link is heating due to optical absorption resulting from imperfections in the devices. When the device temperature gets too high, the transmission must be paused to avoid the fragile quantum signals from being lost, which drastically limits the link's communication rate.
Unique design and correct acoustic wavelength may address the problem
Now a research team from Chalmers University of Technology has come one step closer to a solution. At the center of the link is a nanoscale component, a so-called optomechanical crystal, which is used in the transmission process. In the transmission, light flows through the component whose structure needs to vibrate for a connection between light and quantum computer’s microwave signals to occur. For the coupling, and thus the transmission, to be as efficient as possible, the mechanical vibrations mediating the transmission need to be isolated to the component to the greatest extent possible. The requirement for mechanical isolation has so far been incompatible with optimal thermal anchoring, which is the basis for the heat problems the devices face. The new results suggest another unexplored path that may break this trade-off between communication rate, efficiency and noise.
The proposed solution is published in the scientific journal Optica and is based on a critical redesign of the optomechanical crystal which is now fully clamped to its substrate instead of conventional designs which are suspended. The new type of design allows a considerably larger anchoring region in the component without significant sacrifice of mechanical isolation. A central part of the solution is using vibrations with short wavelengths, which are less prone to leakage and loss compared to vibrations with longer wavelengths. In addition, having them interact with counter-propagating light signals allows for optomechanical coupling rates close to those of state-of-the-art conventional designs.
“We have built a subsystem of the electro-optical link with properties that match our theory and simulations well. This reduces the risk for overheating at higher light powers, which may lead to a increased communication rate between quantum computers,” says Johan Kolvik.
Remaining challenges are under investigation
The researchers' proposed optomechanical conversion solution is seen as an important step in the realization of interconnected quantum computers with high communication rates. But several challenges remain. The optomechanical component has yet to be studied carefully at millikelvin temperatures where the superconducting quantum circuits operate. Also, the designs and measurements published in Optica only looked at the optomechanical components of the transducer, as this is the part exhibiting most of the heating constraints.
“The team is working hard to demonstrate the interface between the gigahertz acoustics and the microwaves next. A redesign at such a basic level means the team needs to reinvent a host of techniques to co-localize microwaves, acoustics, and light to the same tiny volume on a chip. This has taken conventional suspended optomechanical components over a decade. There are early indications but no guarantee that an equivalent path can be found for the new fully clamped devices, says principal investigator Raphaël Van Laer.
More info:
The research study "Clamped and sideband-resolved silicon optomechanical crystals" is published in the journal Optica
The study was carried out by Johan Kolvik, Paul Burger, Joey Frey, and Raphaël Van Laer, working at the Division of Quantum Technology at the Department of Microtechnology and Nanoscience at Chalmers University of Technology. Johan Kolvik and Paul Burger contributed equally to the study.
Contact:
Johan Kolvik, PhD student at Quantum Technology
kolvik@chalmers.se
- Doctoral Student, Quantum Technology, Microtechnology and Nanoscience