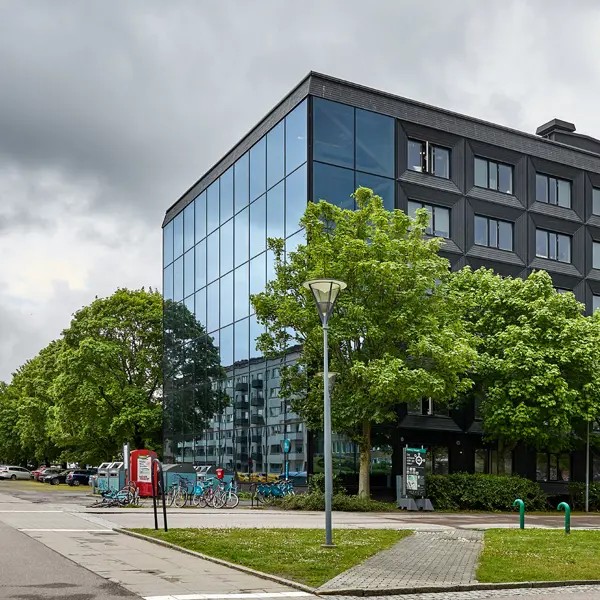
Collaborate in research and innovation
Through its entrepreneurial ecosystem, Chalmers has extensive support to bring research and technology ideas to market.
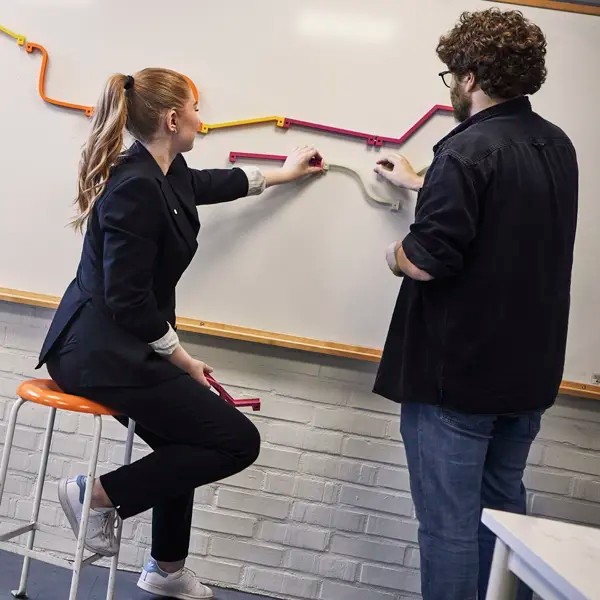
Collaborate in education and with students
We want to give our students the best possible conditions for assimilating the teaching with a clear link between theory and practice. Chalmers therefore collaborates with industry, commerce and society in various ways. One key aspect is to give the education a clear connection with working life.
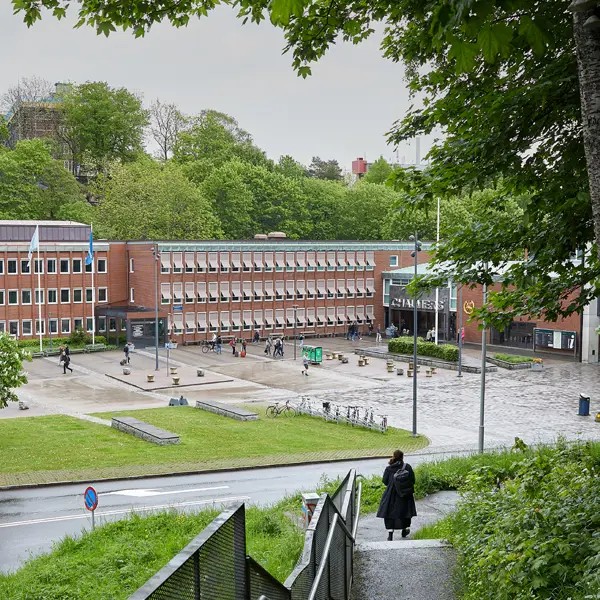
Partnerships and networks
Chalmers collaborates with top universities worldwide and with strategically selected organisations and companies. The partnerships are long-term investments, which run for many years.