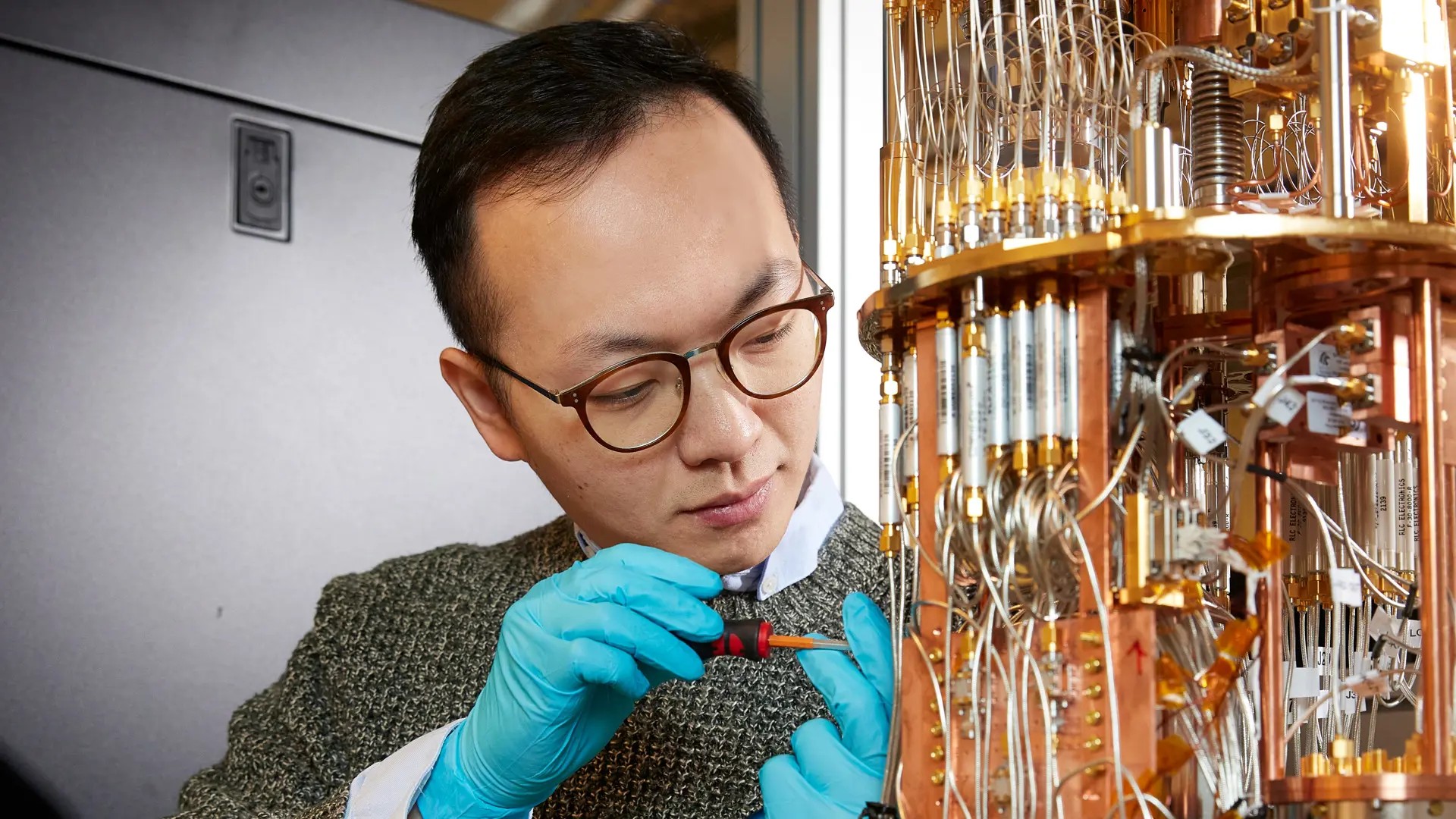
By exploiting the weird phenomena of quantum mechanics, a quantum computer could perform loads of calculations simultaneously – enough to solve problems far beyond the reach of today’s, and tomorrow’s, most capable supercomputers.
The power is in the qubit
The anticipated advantage of quantum computers over regular computers lies in the basic building blocks. In regular computers, the smallest information carrier is the bit which can take either the value 0 or 1. Generally, 1 is represented by an electrical voltage (typically 5 volts) being on, and 0 by no voltage.
By contrast, a quantum computer uses quantum bits – qubits for short – which can be both 0 and 1 at once, due to the quantum property known as superposition.
Because each qubit can represent two values at once, the total number of possible simultaneous states doubles with each added qubit. Two qubits can represent four values at once, three qubits gives eight possible values, and so on. It starts slow, but grows faster and faster. Already 300 qubits could represent more values at once than there are particles in the entire universe. And it only takes 50–60 well-functioning qubits to exceed the computing power in today’s supercomputers.
The story begins
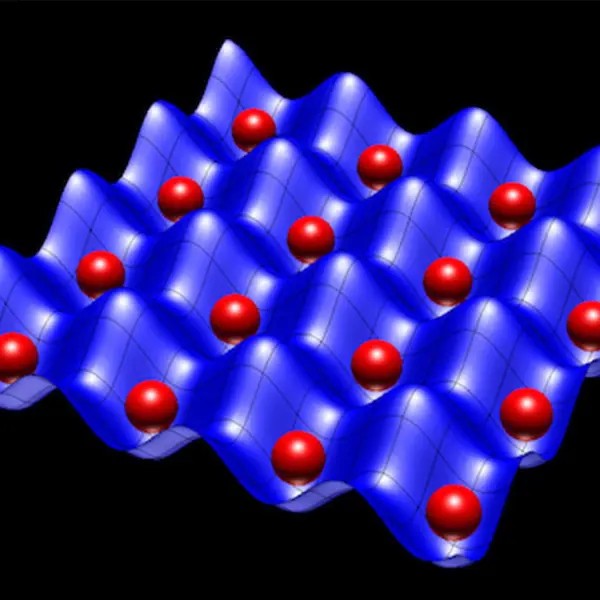
Richard Feynman, the famous physicist, and Yuri Manin came up with the idea of using quantum systems for calculations in the 1980’s. More precisely, they saw that it could be useful for simulations in physics research.
But at that time, nobody knew how to correct the errors that would inevitably occur in a quantum computer and there were no useful algorithms for quantum computers.
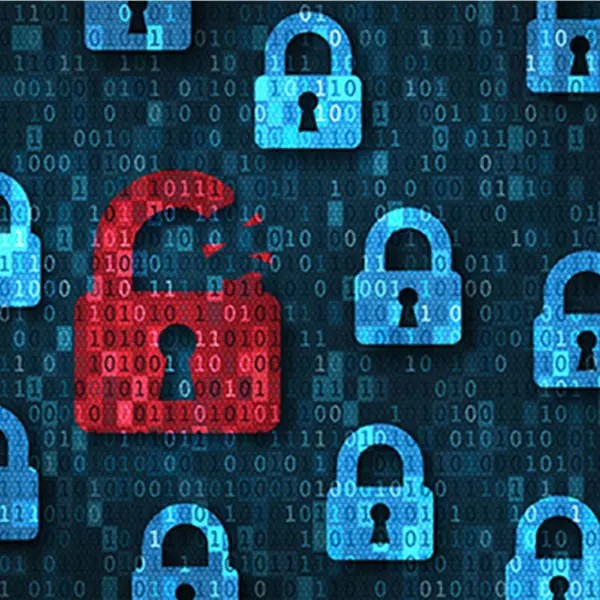
The situation changed drastically in 1994, when mathematician Peter Shor published a quantum algorithm which rapidly finds the prime factors of a given large number – the key to breaking today’s encryption codes. A year later, he showed how a special error correction code can deal with the errors arising in a quantum computer. This sparked a strong interest in realizing a quantum computer. Today, great efforts are made all over the world, at universities as well as companies.
How to build a quantum computer
Unfortunately, there is no simple guide on how to build a quantum computer as it is a very difficult and complex task. But at least, this guide provides a rough overview of the very basics:
1. Select hardware
Pick a controllable quantum system with two (or more) states, for example, an ion having two different energy levels, a superconducting circuit with two different oscillating states, or a tiny semiconductor particle – a quantum dot – with different charge or spin states. Other alternatives are for example, Majorana particles, implanted ions, and photons.
The most promising and developed techniques this far are superconducting circuits and ions. Superconducting circuits are fabricated on a microchip, whereas ions are suspended by electromagnetic fields in a so-called ion trap.
2. Isolate from surroundings
Quantum states are extremely sensitive, and superpositions collapse if they are exposed to disturbances. Therefore, the qubits need to be thoroughly isolated from the surroundings, in order to not “forget” their value immediately. For many types of hardware, this means placing the qubits in an isolated vacuum chamber cooled to just above absolute zero temperature, colder than outer space. Also, take other actions you can think of to reduce disturbances, such as shielding from radiation and magnetic fields.
3. Control the qubits
To make the qubits work for you, you need a way to manipulate them to put them in the desired input states, put pairs of qubits in entanglement (Einstein's "spooky action at a distance"), perform quantum-logic gate operations, and read out the results. In superconducting quantum computers, this is done using microwave pulses. In ion trap computers, it is done using pulses of laser light with specific wavelengths.
4. Improve and practice
Try running a quantum algorithm. Probably, you will find that the qubits forget their values long before the end of the algorithm. Go back to step 1 and try to remove any imperfections in your quantum hardware. Repeat step 2 and take every action you can think of to eliminate disturbances. Then go back to step 3 and remove all possible imperfections in the control equipment. Also, work on increasing the speed in all operations on the qubits. With better qubits and faster operations, you should now be in a better position to run your quantum algorithm.
At the front
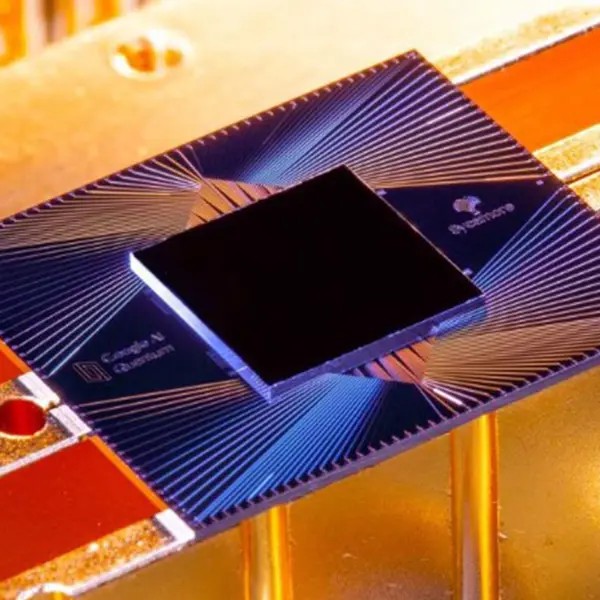
As mentioned before, superconducting circuits and ion traps are the most developed techniques for building a quantum computer.
In October 2019, Google was first to demonstrate a quantum computer solving a problem that is beyond the reach of a regular computer. This is a major milestone in quantum computing, generally referred to as quantum advantage or quantum supremacy. The quantum computer in question was a 53-qubit superconducting device named Sycamore. Read more in Nature and at SVT Nyheter (in Swedish).
Since then, a handful quantum computers have reached quantum advantage. In 2020, Chinese researchers demonstrated quantum advantage with a quantum computer based on light particles, and in 2021, a Chinese team reported having replicated and surpassed Google’s experiment. With their superconducting 60-qubit quantum computer they solved a larger version of the computational task.
In 2022, a photonic quantum computer – named Borealis – of the Canadian company Xanadu proved to computationally beat classical supercomputers at a task called Gaussian boson sampling. In contrast to other quantum computers which had previously attained quantum computational advantage, Borealis is publicly accessible to users via the cloud.
In 2023, IBM demonstrated a quantum computation of the magnetization of a model quantum system by using a 127-qubit superconducting quantum processor, which no classical computer has the memory to simulate. This use of a quantum processor to compute the properties of a real system was advertised as “utility quantum computing” – that is, usefulness even before anyone can demonstrate fault-tolerant, error-corrected quantum computation. WACQT’s Göran Wendin and Jonas Bylander discussed this work in a Nature News & Views commentary. However, just days after IBM’s publication, three papers (1, 2, 3) were uploaded to the Arxiv preprint server with claims of classical simulations outperforming IBM’s results by using better computational methods.
In addition to IBM’s 127-qubit Eagle superconducting processor and Borealis photonics quantum computer, some other quantum computers are available for commercial and research activity via the cloud. For example Rigetti Computing’s 80-qubit superconducting quantum processor, a 23-qubit trapped-ion quantum computer by IonQ, and 256- and 100-qubit neutral-atom quantum computers by QuEra and Pasqal, respectively.
Picture: Google A.I. Quantum’s Sycamore processor. Credit: Erik Lucero/Google
Comparing different quantum computers
News articles often focus on the number of qubits in a quantum computer. However, this number alone tells very little about its performance. There are several other useful metrics to take into account:
- the connectivity between qubits – the number of other qubits that couple to each qubit,
- the types of quantum-logic gates that can be implemented,
- the reliability, often referred to as fidelity, of the gate operations,
- the number of parallel operations that can be implemented, and
- the circuit depth, that is the number of gates in sequence that can be applied to all the qubits before the fidelity has decayed too much during the course of an algorithm.
Coming next
The demonstration of quantum advantage or quantum supremacy, announced in 2019 by Google, is certainly impressive. Their Sycamore quantum processor outperformed a conventional supercomputer in solving a specific problem – but a useless problem chosen only because it is easy on a quantum computer but very hard on a conventional computer.
“The next big milestone in quantum computing is to find a useful problem that is beyond the reach of regular computers, but which a quantum computer with fifty to a hundred qubits can solve. We work intensively on this in collaboration with our industry partners. Probably, it will be within logistics or simulation of large molecules,” says WACQT principal investigator Göran Johansson.
Quantum computers are predicted to be particularly suitable for solving problems that involve a large number of possibilities, such as optimisation problems in logistics or machine learning, and the calculation of properties of large molecules. Breaking today’s encryption codes is further away, since running Shor’s famous algorithm requires thousands of well-functioning qubits.
Quantum computers will most likely be part of hybrid computing systems, where a quantum computer operates as a subroutine or co-processor to a conventional supercomputer. The conventional processors will do most of the work, whereas the quantum processor performs the specific calculations that a quantum computer is significantly better at.
Images:
Optical Lattice, credit: NIST Image Gallery
Google AI Quantum’s Sycamore processor, credit: Erik Lucero/Google
iStock by Getty Images and Chalmers